Key Takeaways:
I. The POSTECH team's new electrolyte achieves an 84.3% energy retention rate after 700 cycles in LLO batteries, a significant improvement over the 37.1% retention observed with conventional electrolytes after just 300 cycles.
II. The new electrolyte actively stabilizes the cathode-electrolyte interface, either by forming a protective layer or modifying the cathode's redox properties, thereby suppressing irreversible oxygen redox reactions.
III. While promising, the commercial viability of this technology hinges on successful scale-up of electrolyte production, cost-effectiveness, and demonstrating a clear advantage over competing battery technologies.
Lithium-ion batteries (LIBs) are the cornerstone of modern portable electronics, electric vehicles, and grid-scale energy storage. However, the quest for higher energy density, longer lifespan, and improved safety continues to drive research into advanced battery materials. Lithium-rich layered oxides (LLOs) have emerged as a promising cathode material, offering a potential 20% increase in energy density compared to conventional nickel-based cathodes. Yet, LLOs face a significant hurdle: the release of oxygen during charge-discharge cycles, leading to capacity fading and voltage decay. A research team at POSTECH has developed a novel electrolyte that effectively suppresses this oxygen release, achieving a remarkable 84.3% energy retention rate after 700 cycles. This breakthrough has the potential to unlock the full potential of LLOs and reshape the landscape of lithium-ion battery technology.
Understanding the Oxygen Release Challenge in LLO Cathodes
Lithium-rich layered oxides (LLOs), with their potential for high energy density, have been a focal point in battery research. However, their inherent instability during charge-discharge cycles poses a significant challenge. The release of oxygen from the cathode structure, driven by irreversible anionic redox reactions, is a primary culprit in this instability. This oxygen release triggers a cascade of detrimental effects, including transition metal migration (especially manganese), structural transformations from layered to spinel-like configurations, and the formation of a resistive cathode-electrolyte interphase (CEI) layer. These processes ultimately lead to capacity fading and voltage decay, limiting the practical lifespan of LLO batteries.
The underlying cause of oxygen release lies in the electronic structure of LLOs and the energetics of the oxygen redox reactions. The d-d Coulomb interaction term (U) and the charge transfer term (Δ) play crucial roles in determining whether the redox reaction will be cationic or anionic. In many LLO materials, U is significantly larger than Δ, favoring the removal of electrons from the unhybridized O 2p states. This leads to irreversible oxygen oxidation and subsequent release as O2 gas. This process is further complicated by structural changes in the LLO lattice, including phase transitions from layered to spinel structures, which hinder lithium-ion diffusion and contribute to performance degradation.
Conventional electrolytes, typically consisting of lithium salts in organic solvents, do little to mitigate the oxygen release problem. They act primarily as passive mediums for lithium-ion transport and do not actively participate in the redox chemistry at the cathode-electrolyte interface. Moreover, these conventional electrolytes are susceptible to parasitic reactions with the released oxygen, leading to the formation of a thick and resistive CEI layer. This CEI layer further impedes lithium-ion transport, exacerbating capacity fade and contributing to the overall decline in battery performance.
The POSTECH team's novel electrolyte represents a significant departure from this conventional approach. While the exact chemical composition remains undisclosed, the reported performance suggests a mechanism that actively interacts with the oxygen redox process. This could involve the formation of a protective layer on the cathode surface, preventing oxygen release, or modifying the redox properties of the cathode material to favor reversible oxygen reactions. The impressive 84.3% capacity retention after 700 cycles strongly indicates the suppression of irreversible oxygen redox, suggesting a more stable cathode-electrolyte interface and improved structural integrity.
Performance Breakthrough: Extended Lifespan and Enhanced Stability
The performance data for the new electrolyte demonstrates a remarkable improvement in battery lifespan. The reported 84.3% energy retention rate after 700 charge-discharge cycles significantly surpasses the performance of conventional electrolytes, which typically show only 37.1% retention after a mere 300 cycles. This translates to a more than twofold increase in cycle life, a crucial factor for applications requiring long-term reliability, such as electric vehicles and grid-scale energy storage. This improvement underscores the effectiveness of the new electrolyte in mitigating the detrimental effects of oxygen release.
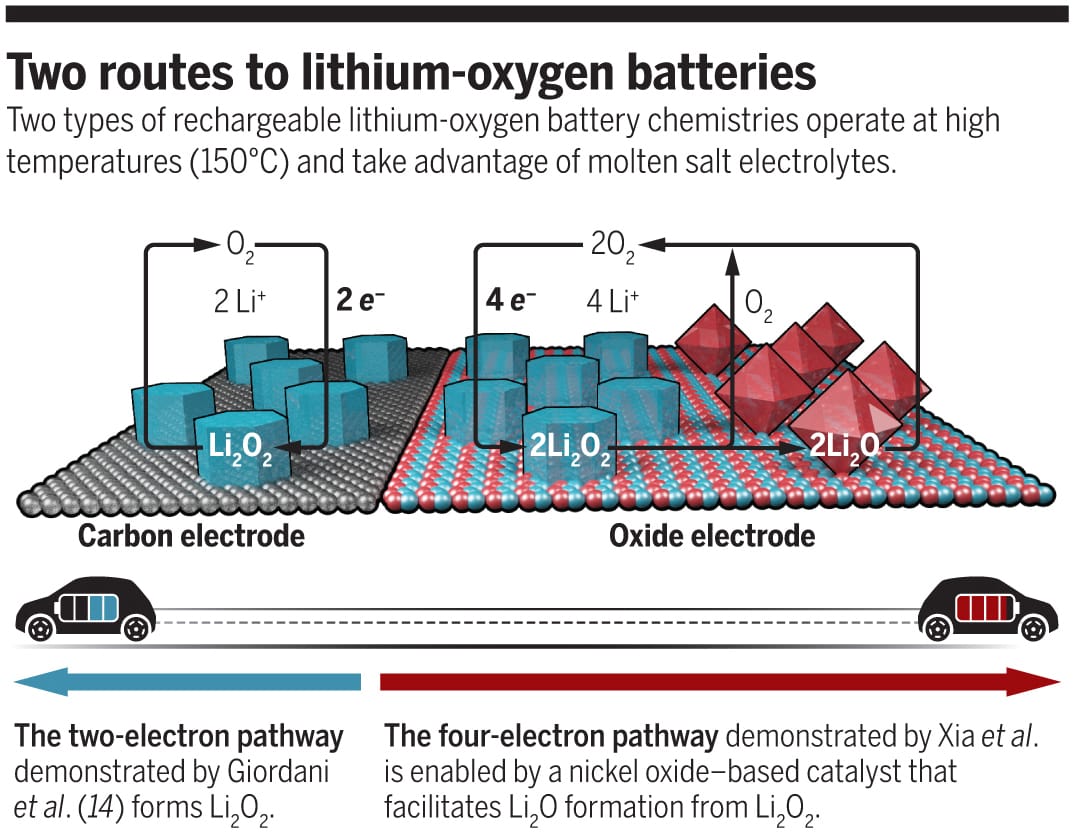
For electric vehicles (EVs), this extended cycle life has significant implications for driving range and cost of ownership. With an 84.3% energy retention rate after 700 cycles, EV batteries using this electrolyte could potentially achieve significantly higher mileage before requiring replacement. This addresses a major consumer concern—range anxiety—and makes EVs more practical for long-distance travel. Furthermore, the reduced frequency of battery replacements translates to lower overall costs, making EVs more economically competitive with conventional gasoline-powered vehicles.
The benefits extend to grid-scale energy storage as well. Longer-lasting batteries are essential for integrating intermittent renewable energy sources, such as solar and wind power, into the electricity grid. The improved cycle life offered by the new electrolyte translates to more reliable and cost-effective energy storage solutions, enabling greater utilization of renewable energy and enhancing grid stability. This advancement could play a significant role in the transition to a more sustainable energy future.
While the initial reports focus on energy retention, the new electrolyte likely also impacts other critical performance metrics, such as Coulombic efficiency and rate capability. A higher Coulombic efficiency indicates a more reversible charge-discharge process, minimizing energy loss during cycling. Improved rate capability allows the battery to deliver higher power output, which is crucial for applications like rapid EV acceleration and responsive grid stabilization. Further research is needed to fully characterize these aspects of the new electrolyte's performance and understand its comprehensive impact on LLO battery behavior.
Scaling Up Production: Challenges and Opportunities
Translating this promising lab-scale result into a commercially viable product requires overcoming significant challenges in scaling up production. The synthesis of the new electrolyte, which likely involves complex chemical processes and specialized equipment, needs to be optimized for large-scale manufacturing while maintaining cost-effectiveness. The availability and cost of raw materials, the environmental impact of production, and the electrolyte's long-term stability during storage and transportation are all critical factors that will determine its commercial success. Competition from established battery manufacturers, particularly those in China with lower production costs, adds another layer of complexity.
Beyond manufacturing, market acceptance and regulatory hurdles pose further challenges. The new electrolyte must compete with existing and emerging battery technologies, including solid-state batteries and other advanced lithium-ion chemistries. Demonstrating a clear advantage in terms of performance, cost, and safety is crucial for gaining market share. Meeting stringent regulatory standards and obtaining necessary certifications for various applications, such as electric vehicles and grid storage, will also be essential for widespread adoption. The rapidly growing battery energy storage system (BESS) market, with its compound annual growth rate of 133% from 2019 to 2023, presents a significant opportunity for this technology, provided these challenges can be successfully addressed. Furthermore, the push for sustainable and ethical sourcing of battery materials adds another dimension to the commercialization landscape. The new electrolyte's composition and manufacturing process must align with these growing environmental and social responsibility concerns to gain widespread acceptance.
The Future of Energy Storage: LLO Batteries and Beyond
The POSTECH team's breakthrough in oxygen suppression represents a significant advancement in the pursuit of high-performance, long-lasting lithium-ion batteries. By addressing the critical challenge of oxygen release in LLO cathodes, this novel electrolyte opens exciting possibilities for a wide range of applications, from powering electric vehicles to enabling more efficient and reliable grid-scale energy storage. While the path to commercialization presents significant challenges, the potential rewards are substantial. Continued research and development, strategic collaborations between academia and industry, and a focus on sustainable manufacturing practices will be crucial for realizing the full transformative potential of this technology and shaping the future of energy storage.
----------
Further Reads
II. Oxygen control retains 84% energy in EV batteries after 700 cycles
III. Advanced Li-ion Battery Technologies 2024-2034: Technologies, Players, Forecasts: IDTechEx